Research
Less than a second after the Big Bang, nature's first liquid was formed- the Quark Gluon Plasma. The Quark Gluon Plasma has some peculiar properties in that it experiences almost no friction (i.e. nearly vanishing viscosity) and still maintains liquid-like properties on scales as small as the size of the proton. The size of the proton is so small compared to every day objects that it's about the same difference as the state of New Jersey compared to the entire Milky Way Galaxy!
​
Since the early 2000's experimentalists have made their own "Little Bang" creators where they can reproduce the Quark Gluon Plasma billions of times, each time with slightly different "knobs" that they can turn to observe its properties underneath different conditions. These experiments are conducted at Brookhaven National Laboratory just down the road from Rutgers University (located on Long Island, NY) and more recently at the Large Hadron Collider at CERN and they are able to reproduce the Quark Gluon Plasma by colliding heavy ions, such as gold or lead ions. The underlying theory of the quarks and gluons that make up the Quark Gluon Plasma is that of Quantum Chromodynamics (QCD). Each individual quark carries a flavor (such as up, down, strange...) and color charge where their strong interactions are mediated by gluons. We are able to understand the thermodynamic properties of the Quark Gluon Plasma by solving QCD on large lattices that run on some of the fastest supercomputers in the world.
To make direct comparisons to experiments, a dynamical description is needed. The components of such a description is shown in the figure below where the key ingredient is the relativistic hydrodynamic description with very small viscosity. The individual components are described in more details below. Also, see my recent talks.
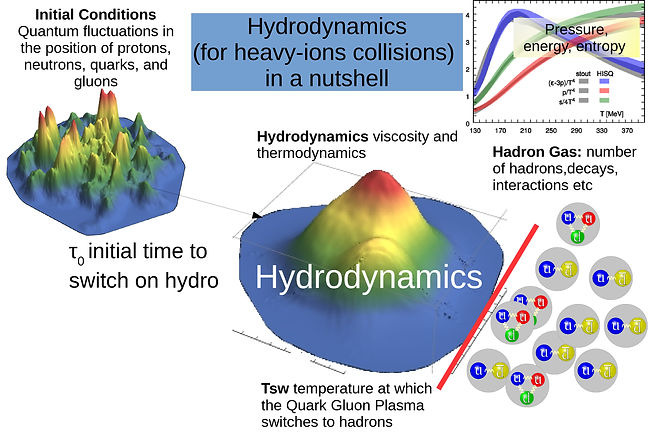

Initial States
Immediately following the collision, the nuclear matter is in a highly chaotic stage where little is known about its properties. In order to study the properties of this initial state one must feed this initial condition into viscous hydrodynamics following by a hadronic afterburner, so it is important that one can disentangle the effects from the initial states vs the following hydrodynamical expansion. To do so, one can compare the initial eccentricities of various initial conditions to the final anisotropic flow harmonics. See more.

Hydrodynamics
What happens when you have a fluid moving close to the speed of light? Relativistic hydrodynamics attempts to answer that question. The Quark Gluon Plasma can be described as a fluid composed of quarks and gluons and it appears that we have created the most perfect fluid, i.e. the complete opposite of tar, in the lab. These studies are done using my event-by-event relativistic viscous hydrodynamic code, v-USPhydro.
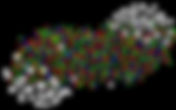
Hadronizaton
Following the Quark Gluon Plasma stage, one must convert the nearly perfect fluid into hadrons suchs as pions and protons. In order to do so, one uses the well-known Cooper Frye freeze-out usually at a chosen constant temperature. When viscosity is considered there are special corrections that need to be considered that can influence the comparison to experimental data. See more and also here.

Jets and Heavy Flavor
Like a bullet shooting through water, fast moving particle(s) lose energy as they pass through the strongly interacting, dense liquid. While we can't actually shoot a bullet through the Quark Gluon Plasma, we can look at particles that are produced very early on during the initial condition. QCD tells us that a hard scattering can produce back to back jets. If these jets are produced at the edge of the Quark Gluon Plasma, one will safely escape whereas the other will get bounced around while having to travel across the entire medium so it gets "lost" among the chaos. My interests involves the connection between fluids and jets.
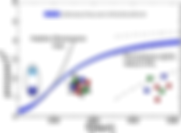
Equation of State
Using Lattice QCD, one can calculation the thermodynamic properties of the Quark Gluon Plasma such as the energy, density, and pressure. Feeding this Equation of State into hydrodynamic calculations, we can determine the temperature reached in heavy-ion collisions (~10^12 Kelvin). These are the highest temperatures reached in Earth and for that honor they made it into the Guinnes Book of World Records. My interest in Lattice QCD involves strange particles and the critical point.
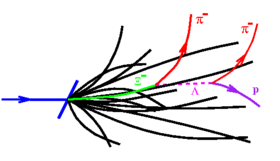
Hadronic Interactions
Due to the high temperatures in heavy ion collisions, hadrons quickly interact and reach chemical equilibrium, followed by kinetic equilibrium. It may be that very heavy, yet-to-be-measured hadrons - may drive these chemical reactions. My research has found that these hadrons could influence a number of experimental signatures for the Quark Gluon Plasma. For instance, the proton/pion ratio, transport coefficients, and the hadron yields.